Miguel A. Reina, Carlo D. Franco, Alberto Prats-Galino, Fabiola Machés, Andrés López, and Jose A de Andrés
INTRODUCTION
Recent research on the ultrastructure of the human spinal dural sac and its contents has enhanced our understanding of the microstructure of the dura mater, arachnoid layer, trabecular arachnoid, pia mater, and nerve root cuffs. This chapter reviews new and traditional concepts regarding these structures and discusses their possible clinical implications. The distribution of epidural fat and its possible role in disposition and kinetics of neuraxial injections are also discussed.
DURAL SAC
The dural sac surrounds the spinal cord inside the vertebral column. It separates the epidural space from the subarachnoid space, ending at the second sacral vertebra. In an idealized shape, the dural sac is cylindrical with its thickness varying from about 1 mm in the cervical region and becoming gradually thinner as it descends (Figures 1 and 2). In the lumbar region, the thickness of the dural sac reaches 0.3 mm, although measurements taken from anteroposterior or lateral may vary somewhat even at the same vertebral level. The dura mater is the most external layer of the dural sac and is responsible for 90% of its total thickness. This fibrous structure, although permeable, somewhat provides mechanical protection to the spinal cord and its neural elements. The internal 10% of the dural sac is formed by the arachnoid layer, which is a cellular lamina that adds little extra mechanical resistance.
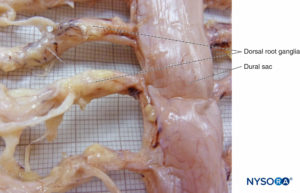
FIGURE 1. Human dural sac. (Reproduced with permission from Reina MA, López García A, de Andrés JA, et al: Thickness variation of the dural sac. Rev Esp Anestesiol Reanim. 1999 Oct;46(8):344-349.)
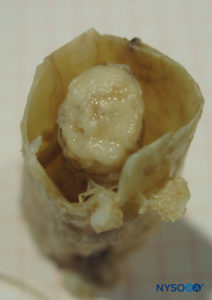
FIGURE 2. Human dural sac and end spinal cord. (Reproduced with permission from Reina MA, Pulido P, López A. El saco dural humano: Rev Arg Anestesiol. 2007; 65:167-184.)
DURA MATER
Dura mater comprises approximately 80 concentric laminas (Figure 3). Each dural lamina has a thickness of approximately 5 μm and consists of thinner laminas containing mostly collagen fibers (Figures 4 and 5). The collagen fibers are oriented in different directions but always within the concentric plane of the dural lamina; hence, they do not cross between the laminas. Each collagen fiber has a smooth surface and measures approximately 0.1 μm (Figure 6). The elastic fibers are fewer, measuring 2 μm in diameter, and have a rougher surface than that of the collagen fibers (Figure 7).
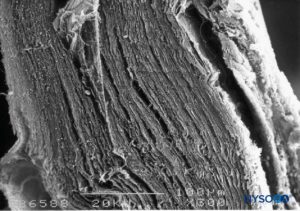
FIGURE 3. Thickness of the dural sac. Scanning electron microscopy. Magnification ×300. (Reproduced with permission from Reina MA, Dittmann M, López A, et al: New perspectives in the microscopic structure of human dura mater in the dorso lumbar region. Reg Anesth. 1997 Mar-Apr;22(2):161-166.)
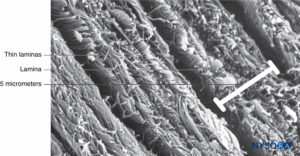
FIGURE 4. Partial thickness of the dural sac. Details of dural laminas. Scanning electron microscopy. Magnification ×4,000. (Reproduced with permission from Reina MA, López A, Dittmann M, et al: Structure of human dura mater thickness by scanning electron microscopy. Rev Esp Anestesiol Reanim. 1996 Apr;43(4):135-137.)
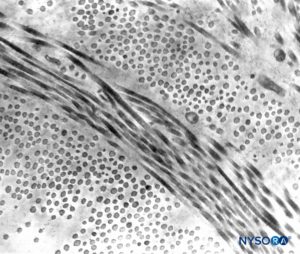
FIGURE 5. Partial thickness of the dural sac. Details of dural laminas. Transmission electron microscopy. Magnification ×20,000. (Reproduced with permission from Raj P: Textbook of Regional Anesthesia. Philadelphia: Churchill Livingstone; 2002.)
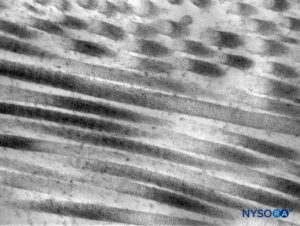
FIGURE 6. Collagen fibers in the thickness of the dural sac. Transmission electron microscopy. Magnification ×50,000. (Reproduced with permission from Raj P: Textbook of Regional Anesthesia. Philadelphia: Churchill Livingstone; 2002.)
Contrary to the classic description of fibers inside dural laminas being arranged longitudinally and parallel to the long axis of the vertebral column, the fibers are actually distributed multidirectionally at random inside each of the concentric dural laminas (Figures 8 to 10). Mastocytes and macrophages are also present within the dura mater (Figures 11 and 12).
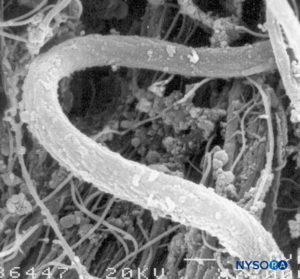
FIGURE 7. Elastic fibers of the dural sac. Scanning electron microscopy. Magnification ×7000. (Reproduced with permission from Reina MA, López A, Dittmann M, et al: External and internal surface of human dura mater by scanning electron microscopy. Rev Esp Anestesiol Reanim. 1996 Apr;43(4):130-4.)
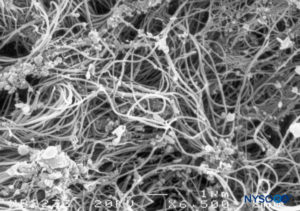
FIGURE 8. Detail of epidural surface of the dural sac. Scanning electron microscopy. Magnification ×6500. (Reproduced with permission from Dittmann M, Reina MA, López A: Neue ergebnisse bei der darstellung der dura mater spinalis mittles rasterelektronenmikroskopie. Anaesthesist. 1998 May;47(5):409-413.)
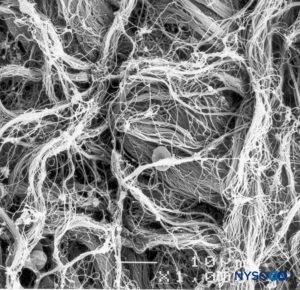
FIGURE 9. Detail of epidural surface of the dural sac. Scanning electron microscopy. Magnification ×1000. (Reproduced with permission from Reina MA, López A, Dittmann M, et al: External and internal surface of human dura mater by scanning electron microscopy. Rev Esp Anestesiol Reanim. 1996 Apr;43(4):130-4.)
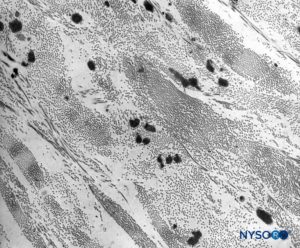
FIGURE 10. Collagen and elastic fibers in the thickness of the dural sac. Transmission electron microscopy. Magnification ×7,000. (Reproduced with permission from Raj P: Textbook of Regional Anesthesia. Philadelphia: Churchill Livingstone; 2002.)
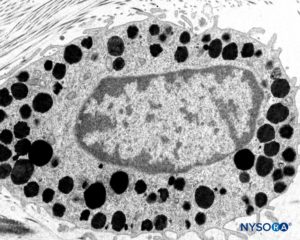
FIGURE 11. Mastocyte in the thickness of the dura mater. Transmission electron microscopy. Magnification ×15,000. (Reproduced with permission from Reina MA, Pulido P, López A. El saco dural humano: Rev Arg Anestesiol. 2007;65:167-184.)
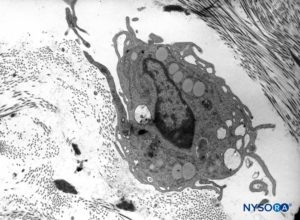
FIGURE 12. Macrophage in the thickness of the dura mater. Transmission electron microscopy. Magnification ×7000. (Reproduced with permission from Raj P: Textbook of Regional Anesthesia. Philadelphia: Churchill Livingstone; 2002.)
ARACHNOID LAYER
Conventionally, the arachnoid layer is described as a fine membrane in close contact with but not adhering to the internal surface of the dura mater. Recent research, however, determined that there is no space between the dura mater and the arachnoid layer (see subdural space). The arachnoid layer is semipermeable and serves as a barrier to limit the passage of substances through the dural sac (Figures 13 and 14). Its thickness is about 50-60 μm (Figures 15 and 16). In its interior, arachnoid cells strongly bond by specific membrane junctions with a thickness of about 10–15 μm. Collagen fibers at the center of the arachnoid layer give strength to the lamina and improve its mechanical resistance. Flat, elongated neurothelial cells occupy the outer portion of the layer. Tearing off the arachnoid layer exposes the subdural space. Neurothelial cells can be found attached either to the internal surface of the dura or to the external surface of the arachnoid layer (Figure 17).
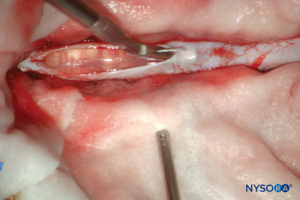
FIGURE 13. Dissection of human dural sac. (Reproduced with permission from Reina MA, Pulido P, López A. El saco dural humano. Rev Arg Anestesiol. 2007; 65:167-184.)
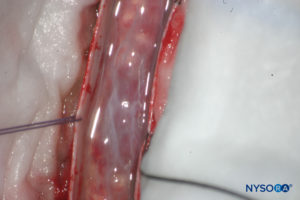
FIGURE 14. Dissection of human dural sac. Dura mater is open; arachnoid layer is closed. (Reproduced with permission from Reina MA, Pulido P, López A. El saco dural humano. Rev Arg Anestesiol. 2007; 65:167-184.)
NEURAXIAL block AND DURAL LESIONS
Piercing the dural sac during a subarachnoid block causes mechanical disruption of both the dura mater and the arachnoid layer. The cross-sectional area of the puncture site produced by a 25-gauge needle is similar regardless of whether the needle has a pencil point or a cutting end. However, the morphology of the lesion varies depending on the design of the needle tip. Pencil-point needles produce a greater and rougher-appearing injury to the dural fibers, while cutting needles produce a U-shaped lesion or flap resembling the open lid of a tin can (Figures 8 to 31).
When using cutting (long bevel) needles, bevel orientation (eg, parallel or perpendicular to the main axis of the cord) does not significantly affect the size or morphology of the lesions in the dura and arachnoid lamina (see Figure 24). The lesion that the needle produces in the dural sac has two components, dural and arachnoid. It is believed that the arachnoid component is vital in limiting cerebrospinal fluid leakage from the subarachnoid space to the epidural space. Therefore, the size and morphology of arachnoid lesions seem to be more important for laminar sealing and cerebrospinal leakage than the size and morphology of dural lacerations.
The incidence of postdural puncture headache (PDPH) has been thought that is affected by the type of needle used (pencil point versus cutting) and of the bevel. The traditional belief that cutting needles result in larger dural lesions (tears) was established in the 1940s and may have been the consequence of the imperfections in the needle design of that era. Modern needles, however, produce clean, U-shaped lesion or flap resembling the open lid of a tin can (see Figure 24).
After needle withdrawal, the U-shaped flap tends to return to its original position due to cerebrospinal fluid pressure and the elastic properties of the dura mater. The dural orifice is almost completely occluded after approximately 15 minutes. On the other hand, lesions produced by pencil-point needles involve a more complex lesion with fiber tearing, sectioning, and separation. The extent of the dural lesion caused by a needle depends on several factors, including the external diameter of the needle, dural and arachnoid sealing mechanisms, needle-tip design, and the quality of needle manufacturing. Needles having the same tip design but different manufacturing methods may not have the same surface quality and may contain microfractures or imperfections, resulting in more or less extensive dural fiber tearing and residual lesions.
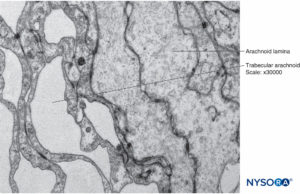
FIGURE 15. Arachnoid cells in the thickness of arachnoid layer. Transmission electron microscopy. Magnification ×40,000.
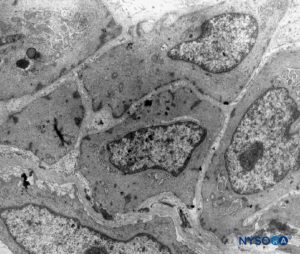
FIGURE 16. Arachnoid cells in the thickness of arachnoid layer. Transmission electron microscopy. Magnification ×4400. (Reproduced with permission from Raj P: Textbook of Regional Anesthesia. Philadelphia: Churchill Livingstone; 2002.)
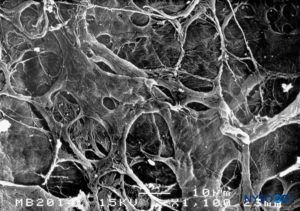
FIGURE 17. Internal surface of dura mater. Scanning electron microscopy. Magnification ×1100. (Reproduced with permission from Reina MA, Dittmann M, López A, et al: New perspectives in the microscopic structure of human dura mater in the dorso lumbar region. Reg Anesth. 1997 Mar-Apr;22(2):161-166.)
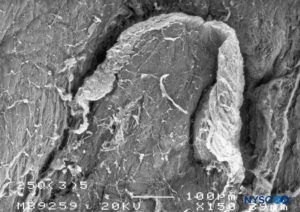
FIGURE 18. Dura-arachnoid lesion produced with 25-G Quincke needle. Epidural surface. Scanning electron microscopy. Magnification ×150. (Reproduced with permission from Reina MA, Castedo J, López A, et al: Cefalea pospuncion dural: Ultraestructura de las lesions durables y abuja’s spindles usages en las punciones lumbers. Rev Arg Anesthesiol. 2008 Jan-Mar 66(1):6-26)
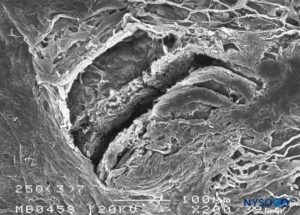
FIGURE 19. Dura-arachnoid lesion produced with 25-G Quincke needle. Arachnoid surface. Scanning electron microscopy. Magnification ×200. (Reproduced with permission from Reina MA, Castedo J, López A. Cefalea pospunción dural: Ultraestructura de las lesiones durales y agujas espinales usadas en las punciones lumbares. Rev Arg Anestesiol. 2008 Jan-Mar 66(1):6-26.)
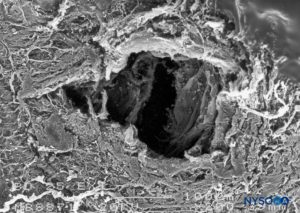
FIGURE 20. Dura-arachnoid lesion produced with 25-G Whitacre needle. Epidural surface. Scanning electron microscopy. Magnification ×200. (Reproduced with permission from Reina MA, López-García A, de Andrés-Ibáñez JA, et al: Electron microscopy of the lesions produced in the human dura mater by Quincke beveled and Whitacre needles. Rev Esp Anestesiol Reanim. 1997 Feb;44(2):56-61.)
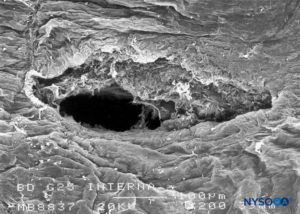
FIGURE 21. Dura-arachnoid lesion produced with 25-G Whitacre needle. Arachnoid surface. Scanning electron microscopy. Magnification ×200. (Reproduced with permission from Reina MA, López-García A, de Andrés-Ibáñez JA, et al: Electron microscopy of the lesions produced in the human dura mater by Quincke beveled and Whitacre needles. Rev Esp Anestesiol Reanim. 1997 Feb;44(2):56-61.)
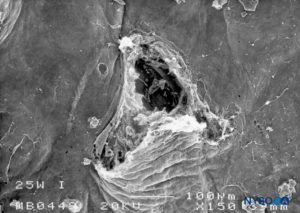
FIGURE 22. Dura-arachnoid lesion produced with 25-G Whitacre needle. Arachnoid surface. Scanning electron microscopy. Magnification ×200. (Reproduced with permission from Reina MA, De Leon Casasola OA, et al: An in vitro study of dural lesions produced by scanning electron microscopy. Reg Anesth Pain Med. 2000 Jul-Aug;25(4):393-402.)
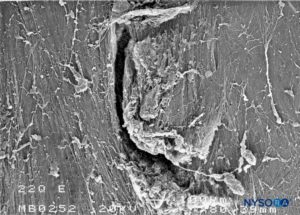
FIGURE 23. Dura-arachnoid lesion produced with 22-G Quincke needle. Epidural surface. Scanning electron microscopy. Magnification ×80. (Reproduced with permission from Reina MA, Castedo J, López A. Cefalea pospunción dural: Ultraestructura de las lesiones durales y agujas espinales usadas en las punciones lumbares. Rev Arg Anestesiol. 2008 Jan-Mar 66(1):6-26.)
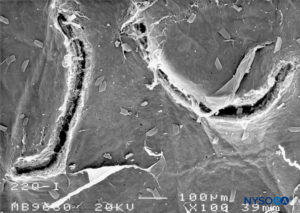
FIGURE 24. Dura-arachnoid lesion produced with 22-G Quincke needle. Arachnoid surface. Scanning electron microscopy. Magnification ×100. (Reproduced with permission from Raj P: Textbook of Regional Anesthesia. Philadelphia: Churchill Livingstone; 2002.)
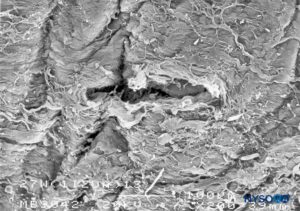
FIGURE 25. Dura-arachnoid lesion produced with 27-G Whitacre needle. Epidural surface. Scanning electron microscopy. Magnification ×200. (Reproduced with permission from Reina MA, Castedo J, López A. Cefalea pospunción dural: Ultraestructura de las lesiones durales y agujas espinales usadas en las punciones lumbares. Rev Arg Anestesiol. 2008 Jan-Mar 66(1):6-26.)
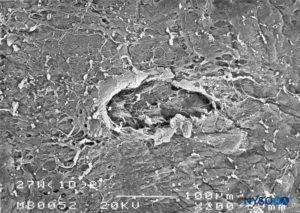
FIGURE 26. Dura-arachnoid lesion produced with 27-G Whitacre needle. Epidural surface. Scanning electron microscopy. Magnification ×200. (Reproduced with permission from Reina MA, Castedo J, López A. Cefalea pospunción dural: Ultraestructura de las lesiones durales y agujas espinales usadas en las punciones lumbares. Rev Arg Anestesiol. 2008 Jan-Mar 66(1):6-26.)
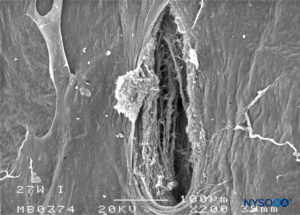
FIGURE 27. Dura-arachnoid lesion produced with 27-G Whitacre needle. Arachnoid surface. Scanning electron microscopy. Magnification ×200. (Reproduced with permission from Reina MA, Castedo J, López A. Cefalea pospunción dural: Ultraestructura de las lesiones durales y agujas espinales usadas en las punciones lumbares. Rev Arg Anestesiol. 2008 Jan-Mar 66(1):6-26.)
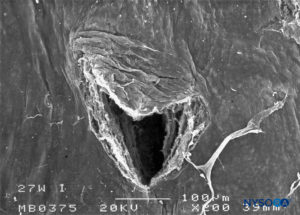
FIGURE 28. Dura-arachnoid lesion produced with 27-G Whitacre needle. Arachnoid surface. Scanning electron microscopy. Magnification ×200. (Reproduced with permission from Reina MA, Castedo J, López A. Cefalea pospunción dural: Ultraestructura de las lesiones durales y agujas espinales usadas en las punciones lumbares. Rev Arg Anestesiol. 2008 Jan-Mar 66(1):6-26.)
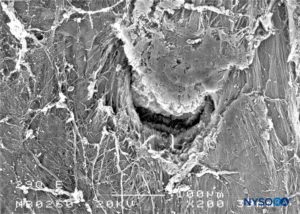
FIGURE 29. Dura-arachnoid lesion produced with 29-G Quincke needle. Epidural surface. Scanning electron microscopy. Magnification ×200. (Reproduced with permission from Reina MA, Castedo J, López A. Cefalea pospunción dural: Ultraestructura de las lesiones durales y agujas espinales usadas en las punciones lumbares. Rev Arg Anestesiol. 2008 Jan-Mar 66(1):6-26.)
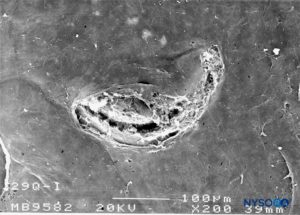
FIGURE 30. Dura-arachnoid lesion produced with 29-G Quincke needle. Arachnoid surface. Scanning electron microscopy. Magnification ×200. (Reproduced with permission from Reina MA, Castedo J, López A. Cefalea pospunción dural: Ultraestructura de las lesiones durales y agujas espinales usadas en las punciones lumbares. Rev Arg Anestesiol. 2008 Jan-Mar 66(1):6-26.
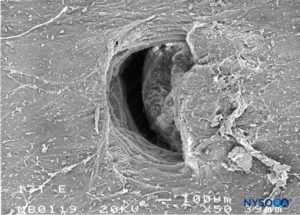
FIGURE 31. Dura-arachnoid lesion produced with 17-G Tuohy needle. Arachnoid surface. Scanning electron microscopy. Magnification ×50. (Reproduced with permission from Reina MA, Castedo J, López A. Cefalea pospunción dural: Ultraestructura de las lesiones durales y agujas espinales usadas en las punciones lumbares. Rev Arg Anestesiol. 2008 Jan-Mar 66(1):6-26.)
Deformation of the spinal needle caused by contacts with vertebral bones or other resistant structures during the neuraxial procedure can also increase the size of the dural lesion. Iatrogenic introduction of skin fragments into the subarachnoid space can also occur.
POSTDURAL PUNCTURE HEADACHE AND TYPE OF NEEDLES
The etiology of PDPH is multifactorial. While the specific chapter on PDPH focuses on pathophysiology, prevention, and treatment, this section focuses on anatomical and equipment-related factors that may influence the occurrence and severity of PDPH. Initially, it was thought that pencil-point needles resulted in less-traumatic perforations of the dural sac. As the morphology of dural lesions became better known, other explanations have been proposed. Microscopic studies of lesions produced by spinal needles showed that the pencil-point needles produce a “burst”-type lesion with extensive fiber damage. However, the increased fiber tearing produced by pencil-point needles may promote greater inflammatory response at the edges of the lesion that paradoxically results in earlier occlusion and lower incidence of PDPH. Cutting needles, on the other hand, produce a “cleaner” tear of the dura with less inflammatory response that results in delayed puncture sealing, which could increase the incidence of spinal headache.
A needle tip blunted after colliding against bone can cause more damage to the fibers. Tip deformation depends on the collision angle and force applied. Cutting needles are especially susceptible to deformation of the needle tip after colliding against bone, contrary to pencil-point needles. However, because PDPH studies generally involve many anesthetists and different techniques, the definitive impact of the needle deformation on PDPH is difficult to study and at this time remains only hypothetical.
As mentioned, the dural lesion produced by the spinal needle has two components, a dura mater lesion and an arachnoid-layer lesion. While the external or dural component of the sac provides mechanical resistance, it is not elastic enough to prevent cerebrospinal fluid leakage. In contrast, the internal or arachnoid lesion can retract to close the defect created by introduction of the needle into the subarachnoidal space and prevent the leakage of cerebrospinal fluid. Because the arachnoid component is probably more important in pathophysiology of PDPH than the dural itself, these lesions should be referred to as “dura-arachnoid” lesions.
TRABECULAR ARACHNOID
Arachnoid mater consists of two layers, the trabecular arachnoid and arachnoid layers. The trabecular arachnoid merges with the cellular plane of the pia mater and emits projections to all the structures that cross the subarachnoid space, including blood vessels and nerve roots. The projections that cover the nerve roots are called arachnoid sheaths (Figures 32 to 40).
During movement, these sheaths stabilize and prevent excessive movements of the nerve roots within the dural sac. However, the sheaths confer little mechanical protection against trauma. Characteristics of the arachnoid sheaths in the cauda equina are variable; some are lax, while others are formed by superimposed planes of the same components with a more compact appearance. The thickness of an arachnoid sheath ranges from 10 to 60 μm. In some cases, one or more nerve roots are enveloped by a single arachnoid sheath, and in others, the nerve root has no sheath at all.
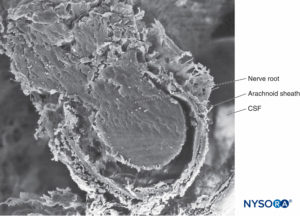
FIGURE 32. Trabecular arachnoid layer. The projections of trabecular arachnoid that cover nerve roots are called arachnoid sheaths. CSF = cerebrospinal fluid. Scanning electron microscopy. Magnification ×100. (Reproduced with permission from Reina MA, López A, De Andrés JA: Hypothesis concerning the anatomical basis of cauda equina syndrome and transient nerve root irritation after spinal anesthesia. Rev Esp Anestesiol Reanim. 1999 Mar;46(3):99-105.)
ARACHNOID SHEATHS OF NERVE ROOTS AND THEIR POTENTIAL ROLE IN NERVE LESIONS
Some cases of cauda equina syndrome and transient neurological syndrome could be explained by the existence of arachnoid sheaths surrounding nerve roots within the dural sac and the fact that needles or (micro)catheters can be inserted into them. An anesthetic solution accidentally injected into the arachnoid sheath of a spinal nerve may not be diluted by surrounding cerebrospinal fluid, thus exposing the nerve root to a higher anesthetic concentration than expected. Consequently, the concentration of the local anesthetic could be magnitudes higher (eg, 20–25 times) in comparison to the concentration of the anesthetic in the rest of the dural sac.
Such high local anesthetic concentration inside the arachnoid sheath could have deleterious effects on nerve roots, as opposed to a typical injection of the same anesthetic solution inside the dural sac but outside the arachnoid sheath. Because it takes time to establish equilibrium inside and outside the sheath, a nerve lesion could develop without direct needle trauma.Injections of local anesthetic through a microcatheter into these arachnoid sheaths could be more devastating than a single injection. This is because the injection of a single large volume would eventually be diluted by leakage outside the sheath, whereas repeated doses of small volumes may be more likely to lead to neurotoxicity due to the continuous or repeated exposure to a high concentration of local anesthetics. Transient root irritation syndrome and cauda equina syndrome may reflect different degrees of nerve damage related to local anesthetic concentration and duration of exposure. Injection of local anesthetic inside arachnoid sheaths in areas close to the spinal cord or to the conus medullaris could affect several nerve roots, while injection in more distal areas may affect single nerve roots.
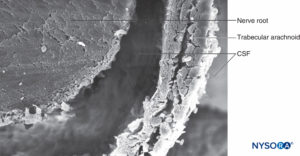
FIGURE 33. Trabecular arachnoid layer. Detail of arachnoid sheaths. CSF = cerebrospinal fluid. Scanning electron microscopy. Magnification x500. (Reproduced with permission from Raj P: Textbook of Regional Anesthesia. Philadelphia: Churchill Livingstone; 2002.)
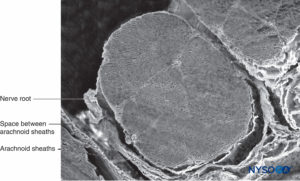
FIGURE 34. Nerve root and arachnoid sheath. Scanning electron microscopy. Magnification ×60. (Reproduced with permission from Torres LM: Textbook of Anesthesia and Pain Management. Aran Ed; 2001.)
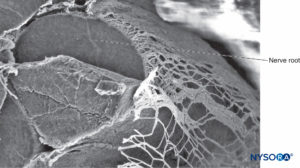
FIGURE 35. Nerve root and arachnoid sheath. Scanning electron microscopy. Magnification ×80. (Reproduced with permission from Reina MA, Villanueva MC, López A: Aracnoides trabecular, piamadre espinal humana y anestesia subaracnoidea, Rev Arg Anestesiol 2008;66: 111–133.)
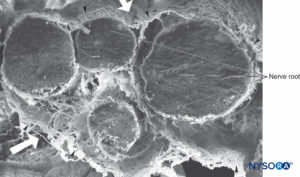
FIGURE 36. Four-nerve root and its arachnoid sheaths. Scanning electron microscopy. Magnification ×100. (Reproduced with permission from Reina MA, López A, De Andrés JA: Hypothesis concerning the anatomical basis of cauda equina syndrome and transient nerve root irritation after spinal anesthesia. Rev Esp Anestesiol Reanim. 1999 Mar;46(3):99-105.)
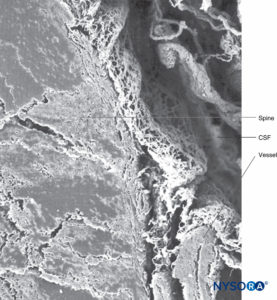
FIGURE 37. Human spinal cord and trabecular arachnoid layer. CSF = cerebrospinal fluid. Scanning electron microscopy. Magnification ×40. (Reproduced with permission from Reina MA, Machés F, López A, et al: The ultrastructure of the spinal arachnoid in humans and its impact on spinal anesthesia, cauda equina syndrome, and transient neurological syndrome. Tech Reg Anesth Pain Management. 2008 July;12(3):153-160.)
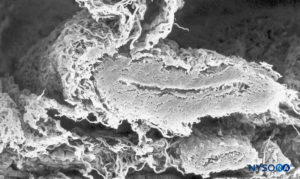
FIGURE 38. Subarachnoid vessel and trabecular arachnoid layer. Scanning electron microscopy. Magnification ×120. (Reproduced with permission from Raj P: Textbook of Regional Anesthesia. Philadelphia: Churchill Livingstone; 2002.)
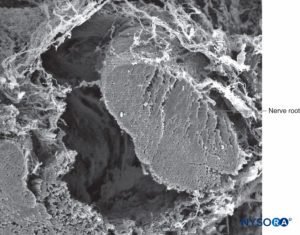
FIGURE 39. Nerve root and arachnoid sheath. Scanning electron microscopy. Magnification ×100. (Reproduced with permission from Torres LM: Textbook of Anesthesia and Pain Management. Aran Ed; 2001.)
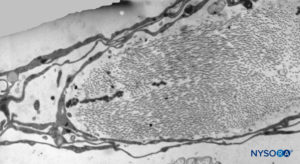
FIGURE 40. Detail of trabecular arachnoid layer. Transmission electron microscopy. Magnification ×5000. (Reproduced with permission from Reina MA, Villanueva MC, López A: Aracnoides trabecular, piamadre espinal humana y anestesia subaracnoidea. Rev Arg Anestesiol. 2008;66:111–133.)
PIA MATER
The structure of the pia mater includes a cellular layer and a subpial compartment (Figures 41 and 42). The cellular layer consists of flat, overlapping pial cells with a smooth and bright appearance (Figure 43). Its thickness is 3 to 5 pial cells (10–15 μm) at the medullary level (Figures 44 to 46) and 2 to 3 cells (3–4 μm) at the nerve root level. Amorphous fundamental substance is found around the pial cells, and the cells measure on average 0.5–1 μm.
The subpial compartment has large amounts of collagen fibers, amorphous fundamental substance, fibroblasts, and a small number of macrophages and blood vessels. The subpial compartment is enclosed between the pial cellular layer and a basal membrane in contact with neuroglial cells.
The subpial compartment from the low thoracic vertebrae has a thickness of 130–200 μm; here, variations in measurements are more significant than in the pial cellular layer (see Figures 41 and 42). At the level of the medullary cone, the thickness of the pia mater is reduced to 80–100 μm; its thickness continues to decrease to merely 50–60 μm in the origins of the cauda equina. At the nerve root level, the thickness of the subpial compartment is 10–12 μm.
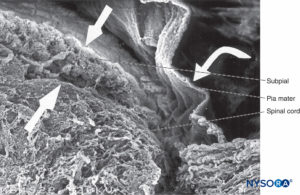
FIGURE 41. Human pia mater and spinal cord. Scanning electron microscopy. Magnification ×70. (Reproduced with permission from Reina MA, De Leon Casasola O, et al: Ultrastructural findings in human spinal pia mater in relation to subarachnoid anesthesia. Anesth Analg. 2004 May;98(5):1479-1485.)
At the level of the medullary cone, there are perforations or circular, ovoid, or elliptical fenestrations over the entire surface of the cellular layer of the pia mater (Figures 47 to 49). While the size of these fenestrations varies, most measure 12–15 μm in length and 4–8 μm in width. At the nerve root level, the pia mater shows similar fenestrations but smaller size (1–4 μm) (Figure 50).Numerous macrophages surround the pial cells. The macrophages differ from pial cells in that they lack long cytoplasmic processes, containing membrane-bound inclusions and a varying number of vacuoles, especially in the peripheral areas of their cytoplasm. The macrophages and other inflammatory cells seen within the pia mater could originate from subpial and subarachnoid blood vessels or from immature pial cells as a result of an unknown stimulus. The fenestrations found in the pia mater appear to be associated with the migration of some immature pial cells as part of an inflammatory response.
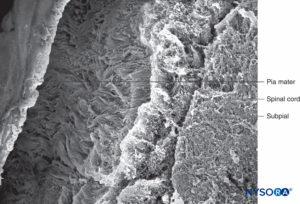
FIGURE 42. Human pia mater and subpial compartment. Scanning electron microscopy. Magnification ×100. (Reproduced with permission from Raj P: Textbook of Regional Anesthesia. Philadelphia: Churchill Livingstone; 2002.)
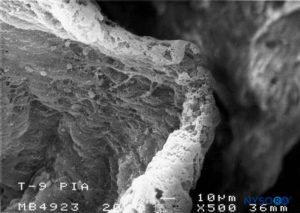
FIGURE 43. Detail of pia mater. Scanning electron microscopy. Magnification ×500. (Reproduced with permission from Reina MA, Wikinski J, De Andrés JA: Una rara complicación de la anestesia epidural y subaracnoidea. Tumores epidermoideos espinales iatrogénicos. Rev Arg Anestesiol. 2008;66:319-336.)
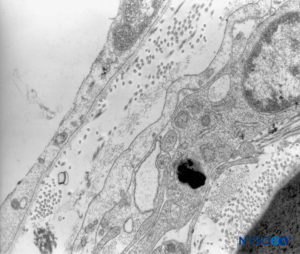
FIGURE 44. Human pia mater. Detail of pial cells. Transmission electron microscopy. Magnification ×12,000. (Reproduced with permission from Reina MA, Wikinski J, De Andrés JA: Una rara complicación de la anestesia epidural y subaracnoidea. Tumores epidermoideos espinales iatrogénicos. Rev Arg Anestesiol. 2008;66:319-336.)
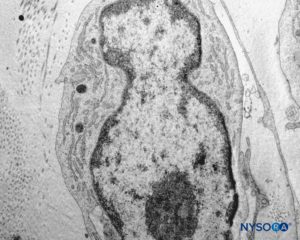
FIGURE 45. Detail of pial cells. Transmission electron microscopy. Magnification ×12,000. (Reproduced with permission from Reina MA, Wikinski J, De Andrés JA: Una rara complicación de la anestesia epidural y subaracnoidea. Tumores epidermoideos espinales iatrogénicos. Rev Arg Anestesiol. 2008;66:319-336.)
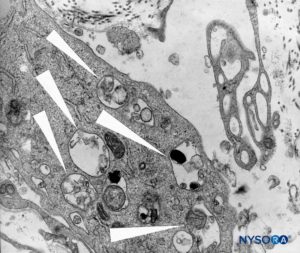
FIGURE 46. Detail of macrophagic cells in spinal pia mater. Transmission electron microscopy. Magnification ×12,000. (Reproduced with permission from Reina MA, Wikinski J, De Andrés JA: Una rara complicación de la anestesia epidural y subaracnoidea. Tumores epidermoideos espinales iatrogénicos. Rev Arg Anestesiol. 2008;66:319-336.)
DURAL SAC LIGAMENTS
The epidural space contains fibrous formations that cross and anchor the dural sac to the vertebral canal. These connective tissue formations are referred to as the anterior, lateral, and posterior meningo-vertebral ligaments (Figures 51 and 52). The anterior meningo-vertebral ligament, which connects the dural sac with the posterior longitudinal ligament of the spine, is more compact. In some patients, fibrous flaps that fix the dural sac to the posterior longitudinal ligament may incompletely divide the anterior epidural space. Anterior ligaments extend from C7 to L5 with a craniocaudal orientation and acquire a transverse orientation at thoracic level T8–9. The length of these ligaments varies from about 0.5 to 29 mm. In the sacral canal, the ligaments thicken to form a perforated medial septum, the “anterior sacral ligament of Trolard.” The lateral meningo-vertebral ligament and the posterior (“Giorda-lengo”) meningo-vertebral ligament are thinner and do not affect the circulation of fluids injected in the epidural space. The “plica mediana dorsalis” is a longitudinal and discontinuous fibrous structure that can be found in the midsagittal region along the posterior epidural space, particularly in the lumbar region.
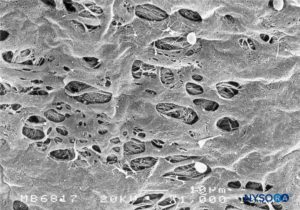
FIGURE 47. Fenestrations in human pia mater of conus medullaris. Scanning electron microscopy. Magnification ×1000. (Reproduced with permission from Reina MA, López García A, de Andrés JA: Anatomical description of a natural perforation present in the human lumbar pia mater. Rev Esp Anestesiol Reanim. 1998;45:4-7.)
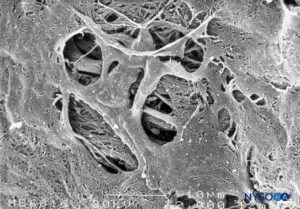
FIGURE 48. Fenestrations in human pia mater of conus medullaris. Scanning electron microscopy. Magnification ×2000. (Reproduced with permission from Reina MA, López García A, de Andrés JA: Anatomical description of a natural perforation present in the human lumbar pia mater. Rev Esp Anestesiol Reanim. 1998;45:4-7.)
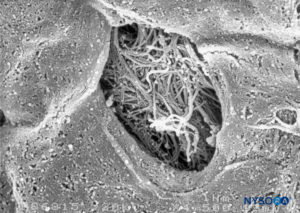
FIGURE 49. Detail of fenestration in human pia mater. Scanning electron microscopy. Magnification ×1500. (Reproduced with permission from Reina MA, López García A, de Andrés JA: Anatomical description of a natural perforation present in the human lumbar pia mater. Rev Esp Anestesiol Reanim. 1998;45:4-7.)
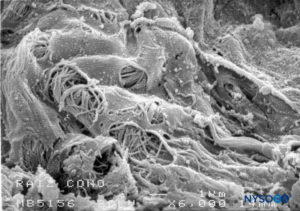
FIGURE 50. Fenestrations in human pia mater of nerve root. Scanning electron microscopy. Magnification ×6000. (Reproduced with permission from Reina MA, Villanueva MC, López A: Aracnoides trabecular, piamadre espinal humana y anestesia subaracnoidea. Rev Arg Anestesiol. 2008;66:111–133.)
EPIDURAL FAT
Epidural fat extends laterally toward the site where articular facets and ligamentum flavum meet. Located between the vertebral arches and the intervertebral foramina, fat wraps around nerve roots within the dural sleeves but without adhering to them. This allows displacement of the dura within the vertebral canal during flexion/extension. Epidural fat does adhere in the midline posteriorly by a vascular pedicle at a point where the right and left portions of the ligamentum flavum meet. The amount of posterior epidural fat increases caudally, from L1–2 to L4–5, and can reach 16–25 mm. Its width also increases in the craniocaudal direction from 6 mm at the L1–2 interspace to 13 mm in the L4–5 interspace. The pedicle of the posterior epidural fat corresponds topographically with the plica mediana dorsalis.
Epidural fat deposits are in contact with the posterior surface of the dural sac and the vertebral lamina but adhere only to the vascular pedicle. Regarding the posterior, epidural fat is homogeneous and is not separated by fibrous septae; laterally, epidural fat appears divided. Sometimes, a septal plane extends between the nerve root exit at the vertebral lamina and the pos-terior longitudinal ligament. Looking at the anterior, dura mater joins the vertebral canal at the height of the disks. It is in this anterior epidural region where the anterior venous vessels are found.
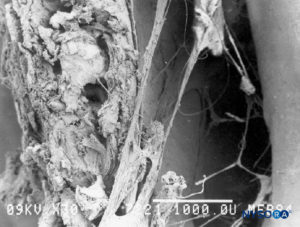
FIGURE 51. Epidural space. Fibrous formations that cross the epidural space. Scanning electron microscopy. Magnification ×30. (Reproduced with permission from Reina MA, Pulido P, López A. El saco dural humano. Rev Arg Anestesiol. 2007;65:167-184.)
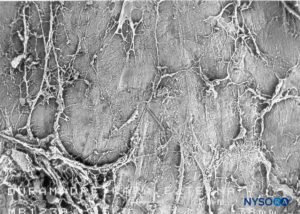
FIGURE 51. Epidural space. Fibrous formations that cross the epidural space. Scanning electron microscopy. Magnification ×30. (Reproduced with permission from Reina MA, Pulido P, López A. El saco dural humano. Rev Arg Anestesiol. 2007;65:167-184.)
CHARACTERISTICS OF THE EPIDURAL FAT IN THE CERVICAL, THORACIC, LUMBAR, AND SACRAL REGIONS
The distribution of epidural fat is variable along the spinal canal, but it tends to be more consistent at different vertebral levels. For example, at the cervical level, adipose tissue is absent or almost nonexistent and sometimes forms a small posterior deposit seen with magnetic resonance imaging axial sections (C7 to T1), with increased signal intensity on T1-weighted sequences. Epidural fat is not generally found in the anterior and lateral regions. At the thoracic level, epidural fat forms a broad posterior band with “indentations.” This band is thicker around the intervertebral space and around the intervertebral disk, becoming thinner at the level of the vertebral bodies and close to the base of the spinal processes of each vertebra. In the middle-upper thoracic region (T1–7), epidural fat follows a continuous pattern with more evident indentations, while in the lower thoracic region (T8–12) epidural fat becomes discontinuous.
At the lumbar level, epidural fat in the anterior and posterior epidural spaces remains separate. The posterior epidural fat is more prominent around the disks of L3–4 and L4–5. In some patients, the posterior epidural fat is cone shaped, with the apex located at the posterior. The thickness of the epidural fat in the lower lumbar zone occupies about 32% of the cross-sectional diameter of the vertebral canal.Below L4–5, the dural sac ends and the sacral canal begins. Here, nerve roots are enveloped by dural sleeves, and epidural fat is the main component within the sleeves.
The morphology and distribution of epidural fat can be altered in pathological conditions. Epidural lipomatosis, for example, is characterized by an increase in epidural fat volume. Excessive fat deposits around the dural sac can cause spinal cord or nerve root compression, leading to neurological symptoms. In kyphoscoliosis, epidural fat is distributed asymmetrically and adipose tissue predominates in the concave portion of the curvature, while the spinal cord is displaced against the vertebral arch. In patients with spinal canal stenosis, epidural fat is characteristically absent or markedly reduced around the stenotic zone.
EPIDURAL FAT AND PHARMACOKINETICS OF EPDIURAL INJECTATES
The distribution of epidural fat in the lumbar vertebral canal is uneven, being more abundant in the dorsal region than in the ventral and lateral regions. The total amount, distribution, and morphology of fat in the epidural space and nerve root cuffs affect the diffusion of substances across these compartments.
Changes in the amount of epidural fat during pathological processes may alter absorption of drugs during epidural block. However, even in the absence of pathological processes, local variations in the amount of fat within the lumbar spinal canal could alter drug kinetics. It is possible that variations in the distance between fat and neighboring nerve tissues affect the disposition of drugs injected and kinetics of the lipophilic drugs. At present, however, the impact of the ultrastructure of epidural and nerve root cuffs on drug kinetics during epidural injection remains unclear.
SUBDURAL SPACE
In contrast to the classical description of a “subdural space” between the dura mater and the arachnoid dorsalis, studies have shown the presence of a solid but delicate tissue composed of specialized neurothelial cells (Figure 53). Neurothelial cells are also referred to as dural border cells. These elongated, fusi-form cells with branched extensions are fragile and scantly cohesive to each other (Figures 54 and 55). Intercellular junctions between neurothelial cells are most susceptible to tearing, and cellular fragments may be seen next to torn neuro-thelial cells (Figures 56 and 57). When tearing occurs along the subdural compartment, small fissures merge into larger ones. Weak cohesive forces between neurothelial cells and lack of collagen fibers facilitate the widening of a fissure, producing the impression of a subdural space. Thus, the classic subdural space appears to be an iatrogenic artifact.
Studying the structure of the subdural compartment may shed light on the origin of cranial and spinal subdural hemato-mas associated with cerebrospinal fluid hypotension.
Subdural anesthetic block, caused by inadvertent injection of local anesthetic partially or entirely between the dura and arachnoid, results in highly unpredictable spinal or epidural anesthesia and complications due to an unanticipated high-level block. Dissection of the weak intercellular junctions between neurothelial cells may allow injected fluids to accumulate in the subdural space. Extent of the subdural block is unpredictable as it depends on the volume of local anesthetic injected and the nature of the dissection (cephalic or circumferential). If the dissection is mainly cephalad, only a few milliliters of anesthetic solution can block cardiorespiratory symptoms.
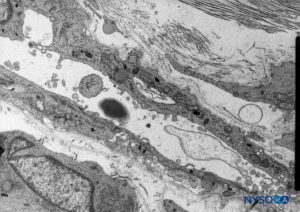
FIGURE 53. Neurothelial cells in the subdural compartment. Transmission electron microscopy. Magnification ×5000. (Reproduced with permission from Reina MA, De León Casasola OA, et al: The origin of the spinal subdural space. Ultrastructure finding. Anesth Analg. 2002 Apr;94(4):991-995.)
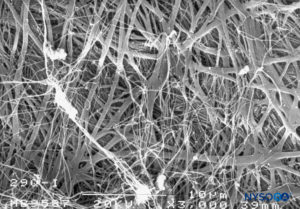
FIGURE 54. Neurothelial cells in the subdural compartment. Scanning electron microscopy. Magnification ×3000. (Reproduced with permission from Reina MA, De León Casasola OA, et al: The origin of the spinal subdural space. Ultrastructure finding. Anesth Analg. 2002 Apr;94(4):991-995.)
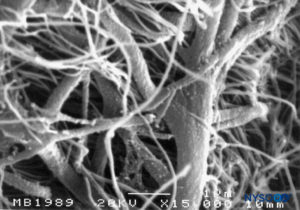
FIGURE 54. Neurothelial cells in the subdural compartment. Scanning electron microscopy. Magnification ×3000. (Reproduced with permission from Reina MA, De León Casasola OA, et al: The origin of the spinal subdural space. Ultrastructure finding. Anesth Analg. 2002 Apr;94(4):991-995.)
NERVE ROOT CUFFS
Bilateral projections of the dural sac (matter) onto the nerve roots form the nerve root cuffs or dural sleeves (Figure 58). Lateral extensions of the dura mater and the arachnoid layer surround the nerve roots as they exit the vertebral canal. The dural sac may contain a certain amount of cerebrospinal fluid around the nerve root. Nerve root cuffs (sleeves) have internal cellular and external fibrillar components (Figure 59). Leptomeningeal cells, similar in nature to arachnoid or pial cells, form the cellular component of root cuffs. These cells are elongated, wider around the nucleus, stratified and oriented longitudinal to the nerve root axis (Figure 60).
At the preganglion level, the cellular component of a root cuff is 5.8–13 μm thick. These cells have cytoplasmic prolongations that encroach on neighboring cells, leaving little extracellular space. Unions between cell membranes are of the type desmosome and have tight junctions (Figure 61). Cells contain mitochondria in their cytoplasm and rough endoplasmic reticulum. Each cell is about 0.15–0.8 μm thick at both ends and 2.2–4.9 μm at the nucleus. The cellular component is arranged in two concentric layers held apart by collagen fibers.
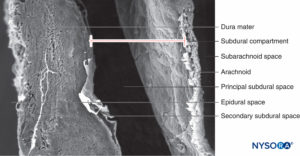
FIGURE 56. Human subdural space in the lumbar meninges. Scanning electron microscopy. Magnification ×180. (Reproduced with permission from Reina MA, López A, De Andrés JA, Villanueva MC, Cortés L: Does the subdural space exist? Rev Esp Anestesiol Reanim. 1998 Nov;45(9):367-376.)
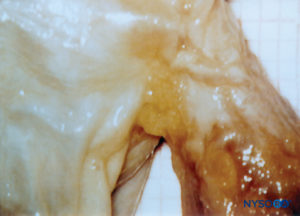
FIGURE 58. Human nerve root cuff. (Reproduced with permission from Reina MA, Villanueva MC, López A, et al: Fat inside the dural sheath of lumbar nerve roots in humans. Rev Esp Anestesiol Reanim. 2007 May;54(5):297-301.)
At the postganglion level, the cellular component has 9–14 single-cell concentric layers and measures 18–50 μm. Their unions are of the desmosome type. The morphology of the cellular component at the ganglion level shows transitional changes while retaining many of the characteristics shown at the postganglion level. The cellular component consists of 25–30 concentric single-cell laminas and has a thickness of 55–60 μm. Ultrastructural aspects of the cellular component at the pre-, post-, and ganglion levels are similar. The cells have rough endoplasmic reticulum widely distributed, and some also contain large vacuoles (0.1 μm) that occupy almost half of the cytoplasmic space. A membranous-like structure found in their cytoplasm may be involved in the production of vesicles (0.05–0.07 μm) needed for pinocytosis. Collagen fibers together with myelinated and unmyelinated axons are seen in the inner side of the cellular plane and are part of the endoneurial fibrillar structure. Specialized membrane unions among cells at the pre-, post-, and ganglion levels ensure a barrier effect, limiting the passage of substances from the epidural space to the nerve axons.
The fibrillar component resides in the outer portion of the root cuff and has a thickness of 100–150 μm (Figure 62). It consists mostly of collagen fibers arranged in concentric laminas with scarce elastic fibers. Large numbers of adipocytes separate dural laminas in groups of three to five concentric layers (Figure 63). Scanning electron microscopy shows adipocytes (Figure 63) extending from the dural sac to the dorsal root ganglia. Adipocytes can be found protruding from inside the wall built by the fibrillar component, out of the external epidural surface of the root cuff (Figures 64 and 65).The fibrillar portion in the dural sac contains about 80 dural laminas with collagen fibers oriented in different directions and few elastic fibers. Its thickness varies between 270 and 350 μm at the lumbar level. Adipocytes are not found within the thickness of the dural sac.
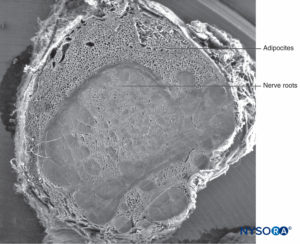
FIGURE 59. Human nerve root cuff. Scanning electron microscopy. Magnification ×12. (Reproduced with permission from Reina MA, Villanueva MC, Machés F, et al: Ultrastructure of human spinal nerve root cuff in lumbar spine. Anesth Analg. 2008 Jan;106(1): 339-344.)
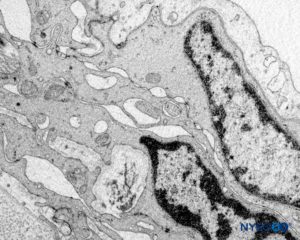
FIGURE 60. Human nerve root cuff. Detail of transition cellular barrier. Transmission electron microscopy. Magnification ×20,000. (Reproduced with permission from Reina MA, Villanueva MC, Machés F, et al: Ultrastructure of human spinal nerve root cuff in lumbar spine. Anesth Analg. 2008 Jan;106(1):339-344.)
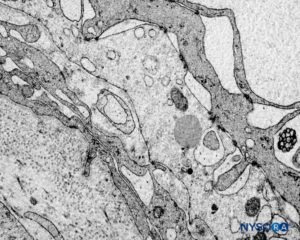
FIGURE 61. Human nerve root cuff. Detail of transition cellular barrier. Transmission electron microscopy. Magnification ×3000. (Reproduced with permission from Reina MA, Machés F, Pulido P, López A, De Andrés JA. Ultrastructure of Human Spinal Meninges. In: Aldrete A. Arachnoiditis, Mexico: Alfil Ed; 2010. pp. 29-46.)
Variations in dural thickness along the dural sac and differences related to the external fibrillar component do not alter the barrier effect, which is the exclusive responsibility of the cellular component.
Scanning electron microscopy has shown that adipocytes measure 50–70 μm and are similar to those found in peripheral nerve samples of the sciatic nerve. The fact that adipocytes appear smaller and lack a spherical shape is most likely due to loss of fat from their vacuoles during sample preparation. Fat in root cuffs covers groups of root axons, although adipocytes are not seen enclosing axons individually. This fat either partially or totally occupies the thickness of the root cuffs’ fibrillar component.
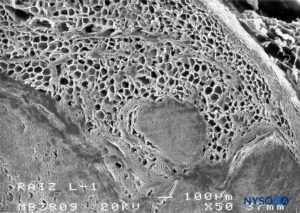
FIGURE 62. Human nerve root cuff. Detail of fat tissue in thickness of nerve root cuff. Scanning electron microscopy. Magnification ×50. (Reproduced with permission from Reina MA, Villanueva MC, López A, et al: Fat inside the dural sheath of lumbar nerve roots in humans. Rev Esp Anestesiol Reanim. 2007 May;54(5):297-301.)
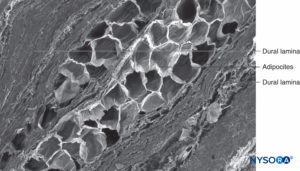
FIGURE 63. Human nerve root cuff. Detail of dural laminas in thickness of nerve root cuff. Scanning electron microscopy. Magnification ×150. (Reproduced with permission from Reina MA, Villanueva MC, Machés F, et al: Ultrastructure of human spinal nerve root cuff in lumbar spine. Anesth Analg. 2008 Jan;106(1):339-344.)
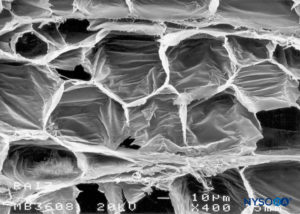
FIGURE 64. Human nerve root cuff. Adipocytes in nerve root cuff. Scanning electron microscopy. Magnification ×400. (Reproduced with permission from Reina MA, Villanueva MC, Machés F, et al: Ultrastructure of human spinal nerve root cuff in lumbar spine. Anesth Analg. 2008 Jan;106(1):339-344.)
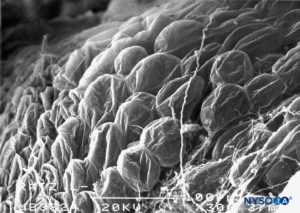
FIGURE 65. Adipocytes on epidural surface of nerve root cuff. Scanning electron microscopy. Magnification ×300. (Reproduced with permission from Reina MA, Villanueva MC, López A, et al: Fat inside the dural sheath of lumbar nerve roots in humans. Rev Esp Anestesiol Reanim. 2007 May;54(5):297-301.)
ADIPOSE TISSUE IN ROOT CUFFS AND DRUG KINETICS
Adipose tissue can be found in the epidural space and inside the nerve root cuffs. Fat in nerve cuffs is in direct contact with nerve root axons and may play a role in the kinetics of lipophilic substances injected near the nerve roots. The small space within the root cuffs and the large amount of drugs available in case of an injection into the cuff may expose the neural elements to a high concentration of local anesthetic as well as a retrograde spread toward the subarachnoidal space.
SUMMARY
This chapter outlined the anatomical characteristics of the neuraxial meninges and related structures and discussed their potential clinical implications.
REFERENCES
- Reina MA, López A, De Andrés JA: Variation of human dura mater thickness [in Spanish]. Rev Esp Anestesiol Reanim 1999;46:344–349.
- Reina MA, Pulido P, López A: El saco dural humano. Rev Arg Anestesiol 2007; 65:167–184.
- Reina MA, López A, Dittmann M, De Andrés JA: Structure of human dura mater thickness by scanning electron microscopy [in Spanish]. Rev Esp Anestesiol Reanim 1996;43:135–137.
- Reina MA, Dittmann M, López A, van Zundert A: New perspectives in the microscopic structure of human dura mater in the dorso lumbar region. Reg Anesth 1997;22:161–166.
- Dittmann M, Reina MA, López A: Neue ergebnisse bei der darstellung der dura mater spinalis mittles rasterelektronenmikroskopie. Anaesthesist 1998;47:409–413.
- Reina MA, López A, Dittmann M, De Andrés JA: External and internal surface of human dura mater by scanning electron microscopy [in Spanish]. Rev Esp Anestesiol Reanim 1996;43:130–134.
- Reina MA, Villanueva MC, López A: Aracnoides trabecular, piamadre espinal humana y anestesia subaracnoidea. Rev Arg Anestesiol 2008;66: 111–133.
- Reina MA, De León Casasola OA, López A, De Andrés JA, Mora M, Fernández A: The origin of the spinal subdural space. Ultrastructure finding. Anesth Analg 2002;94:991–995.
- Reina MA, López A, De Andrés JA, Villanueva MC, Cortés L: ¿Subdural space exists? [in Spanish]. Rev Esp Anestesiol Reanim 1998;45:367–376.
- Reina MA, De León Casasola OA, López A, De Andrés JA, Martín S, Mora M: An in vitro study of dural lesions produced by 25 gauge Quincke and Whitacre needles evaluated by scanning electron microscopy. Reg Anesth Pain Med 2000;25:393–402.
- Reina MA, López A, Badorrey V, De Andrés JA, Martín S: Dura-arachnoids lesions produced by 22G Quincke spinal needles during a lumbar puncture. J Neurol Neurosurg Psychiatry 2004;75:893–897.
- Reina MA, López-García A, de Andrés-Ibáñez JA, et al: Electron microscopy of the lesions produced in the human dura mater by Quincke beveled and Whitacre needles [in Spanish]. Rev Esp Anestesiol Reanim 1997;44:56–61.
- Jokinen MJ, Pitkänen MT, Lehtonen E: Deformed spinal needle tips and associated dural perforations examined by scanning electrón microscopy. Acta Anaesthesiol Scand 1996;40:687–690.
- Puolakka R, Andersson LC, Rosenberg PH: Microscopic analysis of three different spinal needle tips after experimental subarachnoid puncture. Reg Anesth Pain Med 2000;25:163–169.
- Benham M: Spinal needle damage during routine clinical practice. Anaesthesia 1996;51:843–845.
- Reina MA, López A, Manzarbeitia F, Amador V, Goxencia I, Olmedilla MC: Skin fragments carried by spinal needles in cadavers [in Spanish]. Rev Esp Anestesiol Reanim 1995;42:383–385.
- Reina MA, López-García A, Dittmann M, de Andrés JA, Blázquez MG: Iatrogenic spinal epidermoid tumors. A late complication of spinal puncture [in Spanish]. Rev Esp Anestesiol Reanim 1996;43:142–146.
- Reina MA, Wikinski J, De Andrés JA: Una rara complicación de la anestesia epidural y subaracnoidea. Tumores epidermoideos espinales iatrogénicos. Rev Arg Anestesiol 2008; 66:319–336.
- Reina MA, Castedo J, López A: Cefalea pospunción dural: Ultraestructura de las lesiones durales y agujas espinales usadas en las punciones lumbares. Rev Arg Anestesiol 2008;66:6–26.
- Reina MA, López A, De Andrés JA: Hypothesis on the anatomical bases of cauda equine syndrome and transitory radicular irritation syndrome post spinal anesthesia [in Spanish]. Rev Esp Anestesiol Reanim 1999;46:99–105.
- Reina MA, Machés F, López A, De Andrés JA: The ultrastructure of the spinal arachnoid in humans and its impact on spinal anesthesia, cauda equina syndrome and transient neurological síndrome. Tech Reg Anesth Pain Management 2008;12:153–160.
- Reina MA, De Leon Casasola O, Villanueva MC, López A, Maches F, De Andrés JA: Ultrastructural findings in human spinal pia mater in relation to subarachnoid anesthesia. Anesth Analg 2004;98:1479–1485.
- Reina MA, López García A, de Andrés JA: Anatomical description of a natural perforation present in the human lumbar pia mater [in Spanish]. Rev Esp Anestesiol Reanim 1998;45:4–7.
- Luyendijk W: The plica mediana dorsalis of the dura mater and its relation to lumbar peridurography. Neuroradiology 1976;11:147–149.
- Blomberg R: The dorsomedian connective tissue band in the lumbar epidural space of humans: An anatomical study using epiduroscopy in autopsy cases. Anesth Analg 1986;65:747–752.
- Reina MA, Pulido P, Castedo J, Villanueva MC, López A, De Sola R: Characteristics and distribution of the epidural fat [in Spanish]. Rev Esp Anestesiol Reanim 2006;53: 315–324.
- Reina MA, Pulido P, Castedo J, Villanueva MC, López A, De Andrés JA: The epidural fat in different diseases. Contributions of magnetic resonance imaging and possible implications in the epidural and spinal anesthesia [in Spanish]. Rev Esp Anestesiol Reanim 2007;54:173–178.
- Reina MA, López A, Benito-Leon J, Pulido P, María F: Intracraneal and spinal hematoma, a rare complication of epidural and spinal anesthesia [in Spanish]. Rev Esp Anestesiol Reanim 2004;51:28–39.
- Reina MA, López A, Maches F, De Andrés JA: Origin of spinal subdural hematoma [in Spanish]. Rev Esp Anestesiol Reanim 2004;51:240–246.
- Reina MA, Villanueva MC, Machés F, Carrera A, López A, De Andrés JA: Ultrastructure of human spinal nerve root cuff in lumbar spine. Anesth Analg 2008;106:339–344.
- Reina MA, De Andrés J, Machés F, López A: New insights of human spinal root cuffs. In van Zundert A: Highlights in Pain, Therapy and Regional Anaesthesia, 16th ed. Permanyer, 2007, pp 163–173.
- Reina MA, Villanueva MC, López A, De Andrés JA: Fat inside the dural sheath of lumbar nerve roots in human [in Spanish]. Rev Esp Anestesiol Reanim 2007;54:169–172.
- Reina MA, López A, De Andrés JA: Adipose tissue within peripheral nerves. Study of the human sciatic nerve [in Spanish]. Rev Esp Anestesiol Reanim 2002;49:397–402.
- Reina MA, Machés F, Pulido P, López A, De Andrés JA: Ultrastructure of human spinal meninges. In Aldrete A: Arachnoiditis. Alfil Ed, 2010, pp 29–246.
- Reina MA. Atlas of Functional Anatomy for Regional Anesthesia and Pain Medicine. Human Structure, Ultrastructure and 3D Reconstruction Images. Springer, 2015, pp 705–748.
- Reina MA, De Andrés JA, López A: Subarachnoid and epidural anesthesia. In Raj P: Textbook of Regional Anesthesia. Churchill Livingston, 2002, pp 307–324.
- Reina MA, De Andrés JA, López García A: Anatomy in spinal block [in Spanish]. In Torres LM (ed). Textbook of Anesthesia and Pain Management. Aran Ed, 2001, pp 1135–1155.
- Selander D, Sjöstrand J: Longitudinal spread of intraneurally injected local anesthetics. An experimental study of the initial neural distribution following intraneural injections. Acta Anaesthesiol Scand 1978;22:622–634.
NYSORA Tips
- The shape of the dural sac is cylindrical, and its thick-ness is variable.
- The dura mater is permeable and occupies 90% of the thickness of the dural sac.
- The arachnoid layer is semipermeable and governs the passage of substances.
- Dura-arachnoid lesions can differ depending on the type of spinal needle.
- Pencil-point needles produce a more destructive lesion, while cutting needles produce a U-shaped lesion, although the sizes of both are similar.
- The trabecular arachnoid covers the nerve roots and forms arachnoid sheaths.
- Pia mater has fenestrations at the level of the medullary cone.
- Epidural fat distribution is variable along the spinal canal but is consistent at different vertebral levels.
- Epidural fat volume is increased in epidural lipomatosis, distributed asymmetrically in kyphoscoliosis, and absent in stenosis.
- The “subdural space” is in fact occupied by delicate tis-sue composed of specialized neurothelial cells. The tearing of the subdural compartment gives origin to what we know as the subdural space.
- In root cuffs, there is a cellular component that governs the diffusion of substances. Root cuffs also contain large numbers of adipocytes in their thickness.